|
 |
» Home » Membership » Awards » List of past recipients » CSJ Award » Imanaka
|
|
|
 |
Elucidation of the Molecular Mechanisms involved in Adaptation of Thermophiles to High Temperature Environment
Since the discovery of hyperthermophiles about two decades ago, much attention has been given to how life can deal with high temperature environments. Dr. Tadayuki Imanaka has isolated many hyperthermophilic organisms, and has proceeded to study the thermostability of their proteins, their heat maturation, and the stability of their DNA. His research methodology has covered vast fields, including gene and protein engineering, genetics, molecular and structural biology, and thermodynamics.
|  |
Prof. Tadayuki Imanaka
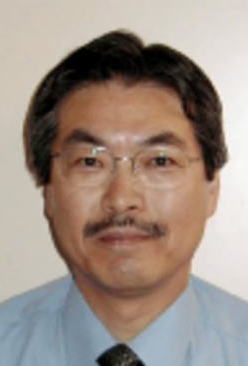 Department of Synthetic Chemistry and Biological Chemistry,
Graduate School of Engineering,
Kyoto University
|
|
1. Molecular mechanisms leading to protein thermostability
The main interactions that govern the three-dimensional structure of a protein are disulfide bonds, ionic interactions, hydrophobic interactions, and hydrogen bonds. Increases in thermostability have been discovered by incorporating additional interactions within a peptide chain, or by intersubunit interactions.
(1) The gene encoding neutral protease (NprT) from the moderate thermophile Bacillus stearothermophilus CU21 was cloned and sequenced. Through site-directed mutagenesis, the Gly141 residue in the α-helix that connects the two domains of the protein was converted to Ala141. This did not lead to a change in activity, but led to an increase in thermostability. By the introduction a single methyl group, this was the first example of stabilizing an enzyme by strengthening hydrophobic interaction and increasing the packing of an α-helix. (2) A hyperthermophilic archaeon, Thermococcus kodakaraensis KOD1, which grows at temperatures up to 100°C, was isolated from a solfatara on Kodakara Island, Kagoshima, Japan. The O6-methylguanine-DNA methyltransferase (MGMT) from T. kodakaraensis was composed of 174 amino acid residues, and functions as a monomer. The protein was crystallized and its three-dimensional structure was determined at 1.8 Å resolution. The bacterial counterpart of this protein in Escherichia coli is the AdaC protein. The structure of this protein was also determined and the structures of two similar proteins with drastic differences in thermostability were compared. When comparing corresponding α-helices, ionic interactions within the helices (intrahelix ion pairs) were only found in MGMT, leading to the stabilization of secondary structure. MGMT also harbored a greater number of ionic interactions between helices (interhelix ion pairs), resulting in the stabilization of tertiary structure. The ΔG of a mutant protein Glu93Ala, designed to disrupt ionic interactions, decreased by a value of approximately 4 kJ/mol. (3) KOD DNA polymerase was crystallized and its three-dimensional structure was determined. The enzyme was disk-shaped (60Å x 80 Å x 100 Å), and two disulfide bridges (Cys428-Cys442, Cys506-Cys509) at the interface of the Fingers domain and Palm domain were found to contribute to the thermostability of this enzyme. (4) The ribulose 1,5-bisphosphate carboxylase/oxygenase from T. kodakaraensis (Tk-Rubisco) increases its stability by taking a unique quaternary structure. By determining the three-dimensional structure of the protein, Tk-Rubisco was found to be a toroid-shaped pentagonal decamer comprised of five L2 dimers. The interface between dimeric structures was mainly composed of ionic interactions involving Glu-63, Arg-66, and Asp-69. Mutant proteins, in which these individual residues, or all three, were replaced by serine, were constructed and analyzed. As a result, the formation of this unique quaternary structure results in a significant increase in thermostability (15°C), enabling the protein to function in a stable manner at 100°C.
2. Heat maturation of proteins from hyperthermophiles
A large number of genes from hyperthermophiles have been cloned, and their protein products have been biochemically examined. While expressing these genes in E. coli, or in other words at low temperatures (30-37°C), a difference in protein conformation was noticed when compared to the native protein produced in the hyperthermophile at high temperature. For example, in the cases of glutamate dehydrogenase or indole pyruvate: ferredoxin oxidoreductase, heat treatment of these recombinant proteins result in conformational changes, leading to structures identical to those of the native proteins. It was clarified that hyperthermophilic proteins produced at lower temperatures are trapped in a semi-stable structure, and heat treatment is necessary for these proteins to efficiently obtain their optimal and stable structures. This phenomenon is now called heat maturation.
3. Molecular mechanism of the thermostability of DNA
Factors that stabilize the DNA of hyperthermophiles include histone-like proteins, potassium ions, polyamines, and reverse gyrase. It was found that the melting temperature of DNA is elevated by over 20°C in the presence of histone-like proteins. Likewise, it has been demonstrated in vitro that polyamines such as spermine and spermidine, as well as high concentrations of potassium ions also repress thermal denaturation of DNA. The hyperthermophile-specific enzyme reverse gyrase is a topoisomerase, and introduces positive supercoils into circular DNA, enhancing the thermal stability of DNA. The presence of this activity has been confirmed in T. kodakaraensis, and by disrupting this gene, he found that the mutant strain lacking reverse gyrase displays a retardation in growth at temperatures above 80°C and cannot grow at 93°C. This clearly displays that reverse gyrase plays an important role in stabilizing DNA at high temperatures.
As described above, the main approach taken by Dr. Tadayuki Imanaka has been to reveal detailed, chemical bases of various intriguing biological phenomena, in other words, a chemical biology approach. His achievements have earned high respect in the international scientific community, and are therefore worthy of receiving the The Chemical Society of Japan Award.
|
|
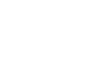 |
|